Evaluation of Size, Membrane Integration and Stability of three-Component Thermo-Sensitive Liposomes as Nanocarriers-Juniper publishers
Global Journal of Nanomedicine-Juniper publishers
Introduction
Drug carrier systems (dendrimers, liposomes, polymers, micelles and metal nanoparticles) are promising tools in the treatment of many diseases by providing decreased drug toxicity to healthy cells and tissues and meanwhile increasing the drug bio-availability in targeted cites [1]. Liposomes are artificially formed vesicles consist of lipids that enclosed as spherical, non- aqueous compartment [2]. Liposomes have been widely used as a model system of a cell membrane, and more recently have been developed for the controlled release of pharmaceuticals and macromolecules. Liposomes can increase the tolerance of active compounds by lowering the active toxicity of its encapsulated contents, [3] whilst also opening up the possibility of transporting pharmaceuticals into the tissue of whim in a controlled manner [4].
After administration to the blood stream, liposomes are mainly taken up in by the liver and spleen [5] thus, it is essential to increase the circulation time of the liposomes in the blood so they can reach the tissues or cells of interest [6]. Improved circulation time can be achieved by designing intelligent liposomes which releases its contents only after they are subjected to triggering conditions, such as instant pH change, hyperthermia, or a magnetic field. Structural properties and characteristics of liposomes (size and lamellarity) can be easily modified by altering their lipid composition and method of preparation [7]. For example, multilamellar vesicles (MLV) can be formed spontaneously when phospholipids are hydrated into aqueous solutions, and large unilamellar vesicles (LUVs) can be prepared from MLVs for example by extrusion or freeze-thawing. On the other hand, preparation of smaller sized, homogenous populations of so called small unilamellar vesicles (SUV) requires -in addition to the thin film preparation method-laborsome size- reducing and homogenization methods (e.g. freeze-thawing, sonication, extrusion, ultracentrifugation) [8] and these time consuming methods of preparation limit the widespread use of liposomes.
Methods for the rapid preparation of homogenous populations of encapsulating trigger-sensitive liposomes are widely sought and would find tremendous application for controlled release. We recently reported on a one-step, ultrarapid (1h) method coined the curvature tuned preparation (CTP), method for the preparation of homogenous encapsulating liposomes, based on a rapid pH jump followed by an equilibration period at a specified temperature, which has been successfully developed using diverse types of phospholipids [9-11].
Lipid assembly to closed vesicles or other types of structures, such as micelles, tubes and planar bilayers is strongly dependent on the lipid geometry, which is defined by the value of critical packing factor, P, which is the ratio of volume occupied by a single lipid molecule to the area occupied by the lipid polar head group and length of the lipid tail [10-12]. Another important property of the lipids is the critical melting temperature (TM) where the lipid phase transition from rigid gel to liquid crystalline phase takes place. External parameters affecting those characteristics (e.g pH, temperature and ionic strength) determine the interaction between the lipids in a synergetic manner with the lipid geometry and the thermal phase behavior of the lipid [13].
The aim of this study is to implement the CTP method for the preparation of thermo-sensitive liposome for the temperature-triggered drug release using hyperthermia. The prepared liposomes were analyzed in terms of their membrane integration by differential scanning calorimetry (DSC), fluorometric measurement of calcein release in the presence of serum, liposome fusion at increasing calcium ions as well as the triggering effect of temperature for controlled release (Structure 1).
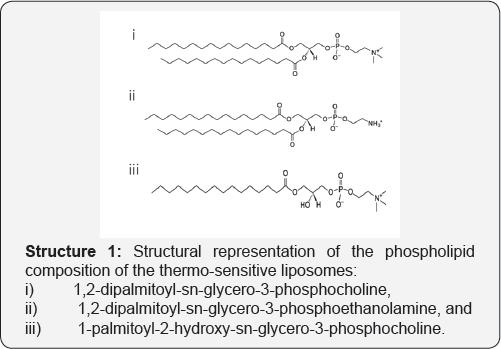
Experimental Section
Materials
Phospholipids were supplied as a powder by Avanti Polar Lipids, Inc. and used without further purification. Sodium hydroxide, hydrochloric acid, di-sodium hydrogen phosphate (anhydrous, reagent grade), Na2HPO4), sodium dihydrogen phosphate (anhydrous), extra pure, (NaH2PO4), calcein and glycerol 99.5%, reagent grade, were purchased from Scharlau Chemie SA. Sodium chloride was provided by Riedel-de Haen. Milli-Q water (1.82 Ω.cm-1) was obtained using a Simplicity 185 Millipore-Water System.
Preparation of encapsulating liposomes
Calcein encapsulating liposomes were prepared via curvature tuned preparation method reported previously. Briefly, 50mg of a phospholipid formulation of 1,2-dipalmitoyl- sn-glycero-3-phosphocholine (DPPC), 1-palmitoyl-2-hydroxy- sn-glycero-3-phosphocholine (lyso-PPC) and phospholipid 1,2-dipalmitoyl-sn-glycero-3-phosphoethanolamine (DPPE) in predetermined molar concentrations were directly rehydrated in a pre-heated calcein mixture in PBS buffer (0.1M, pH 7.4) at 45 °C under argon supply while the mixture was continuously stirred. The solution was then subjected to a rapid pH jump (pH 7.4 ⋄ pH 11 ⋄ pH 7.4] followed by an equilibration period of 25min, where lipid clusters curl into encapsulating liposomes. The resulting liposomes were purified using G25 Sephadex column and stored at 4 °C.
Transmission electron microscopy (TEM) imaging
Using a glass pipette, a drop of sample was added to a 200 mesh copper grid with a thin film of Formvar polymer and carefully dried using filter paper. The sample was then left at room temperature until a dried film was obtained. Transmission Electron Microscopy (TEM) analyses were performed using a JEOL 1011 transmission electron microscope operated at 80 keV with an ultra-high-resolution pole piece providing a point resolution of 2 A°. Micrographs (1024 pixels x 1024 pixels) were acquired using a Mega view III multiscan-CCD camera. Images were analyzed with an iTEM image analysis platform and the mean diameter was calculated measuring at least 100 particles from the series of experiments (n±3).
Differential scanning calorimetry (DSC) studies
All calorimetric scans were performed on a Perkin-Elmer DSC7 operating at a scan rate of 1K/min with a sample mass of approximately 5mg and a reference pan containing an equal mass of buffer. The scan rate was carefully optimized to be the minimum allowable with a sufficient signal-to-noise output. Calibration of the calorimeter was performed prior and subsequent to scanning of the lipid dispersion. Three aliquots from each preparation were scanned and the results averaged. Preparations were repeated and further aliquots scanned to check the reproducibility of the preparation method.
Size and size distribution studies using photon correlation spectroscopy (PCS)
The mean diameter of the liposome emulsions and the size distribution, presented as a function of polydispersity index (PI),were measured using Zeta Sizer 3000H (He-Ne laser (633nm), detector angle of 90o) Malvern Instruments, Inc., which measures the rate of fluctuation of the light scattered from the particles using photon correlation spectroscopy (PCS). Standard deviations were calculated from the mean of the data of a series of experiments (n>3) conducted using the same parameters.
Calcein release
Liposomes were incubated at changing concentrations of liposomes over 24hours at 37 °C, and intermittent measurements of calcein leakage from liposomes were analyzed. The change in fluorescence intensity due to calcein release from the vesicles was monitored with a Cary Eclipse Fluorometer where excitation and emission wavelengths were set at 490 and 520nm, respectively. The amount of calcein released after time (t) was calculated according to:
RF (%) = 100 (It-I0)/(Imax-I0) Eq. (1)
Where Imax is the measured intensity after the Triton X-100 treatment and I0 intensity at time zero.
Results and Discussion
Characterization of liposomes
Thermosensitive liposomes composed of 1,2-dipalmitoyl-sn- glycero-3-phosphocholine (DPPC), 1-palmitoyl-2-hydroxy-sn- glycero-3-phosphocholine (lyso-PPC) and 1,2-dipalmitoyl-sn- glycero-3-phosphoethanolamine (DPPE) (Figure 1). were used to prepare liposomes. The liposome properties were studied at different DPPE concentrations (from 0 to 6 mol%) at a constant percentage of lyso-PPC (12 mol%). During preparation, the temperature was kept constant at 45 °C, which is higher than the melting temperature of DPPC (41 °C). Transmission electron microscopy (TEM) showed DPPE was required for the formation of liposome vesicles (Figure 2). The liposome size decreased from 310 to 80nm when the molar % of DPPE was increased from 1.5 to 6% (Figure 2 & 3). At higher contents of DPPE, due to the truncated cone shape of DPPE [13], the lipid bilayer membrane had a higher curvature, which leads to smaller sized liposomes (Table 1).
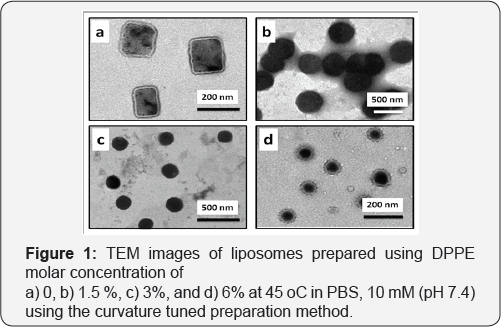
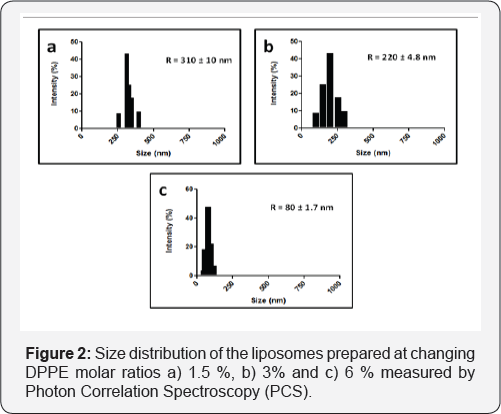
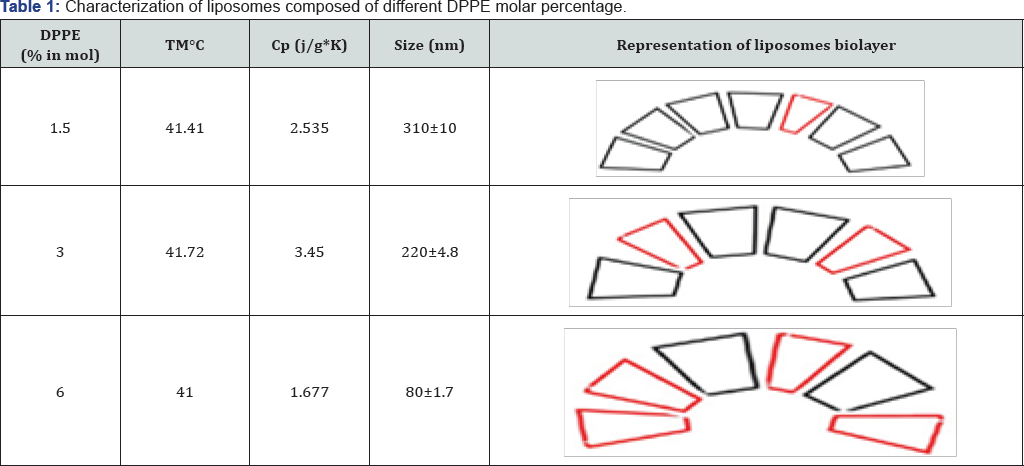
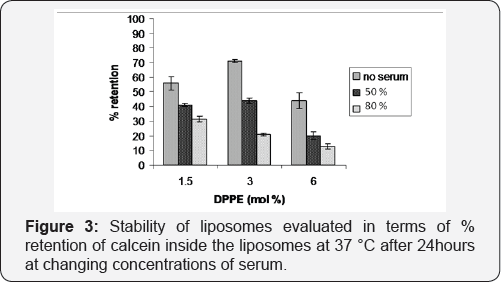
Stability analysis in the presence of serum
The stability of liposomes at different serum concentrations was evaluated in terms of % of the retention of calcein inside the liposomes at 37 °C after 24hour of exposure to serum, for different mol % of DPPE. Supporting the results obtained from DSC, the lipid formulation of 3% DPPE was the most stable when incubated in the absence of serum. In the presence of 50% serum, (approximately equivalent to levels found in blood), the retention is similar slightly higher than with 1.5 mol% of DPPE and clearly higher as compared to that obtained with the 6mol% DPPE formulation. However, when the serum concentration was increased to 80%, the retention of the calcein shows a decrease proportional to the DPPE concentration as at high DPPE contents, there is an increased amine group concentration on the liposome surface, and the attack by serum proteins to these amine groups disturbs the membrane stability. Size analysis with DLS showed that at increasing serum concentrations, liposomes start to form an increasing number of micelles of 8-15 nm, with increasing amounts of DPPE (Figure 4), which can be explained by the charge and phase dependent destruction of the membrane by DPPE.
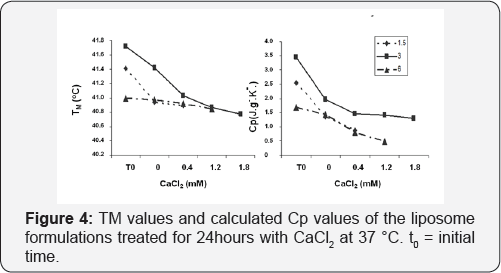
Stability of membrane in presence of Ca2+ ions
The interaction of cations with phospholipid monolayers is of interest, particularly for calcium as this divalent cation plays a special role in biological membranes. In general, calcium induces more crystalline-like phase behavior due to its ability to chelate negatively charged phospholipid head groups [14]. Phosphatidylserine (PS), Phosphatidic acid (PA), and Phosphatidylinositol (PI) containing monolayers are more sensitive to the presence of calcium than monolayers composed of neutrally charged lipids. As well as lateral lipid self-diffusion, calcium ions, affect the rotational diffusion of the lipid head group. Therefore, calcium, as a fusogenic agent, could be expected to have an influence on a phospholipid membrane [15].
To probe the influence of Ca2+ on membrane integration, DSC studies were performed with the samples after 24hours of incubation. Transition peak which is the indicator of a transition from gel to gel crystalline was recorded and corresponding melting temperatures (TM) and specific heat capacitance were calculated. As can be seen from Figure 5, no transition peak was observed at calcium concentrations higher than 0.4mM for 1.5% DPPE and 1.2mM for 6% DPPE. On the other hand, a decrease in Cp starting from 0.4mM CalCl2 was observed for the liposome sample composed of 6% DPPE molar concentrations and corresponding TM values support this observation. At concentrations higher than 0.4mM CaCl2 concentration, the effect of calcium is more discernable, and at higher calcium concentration, the lipid formulation of 3% DPPE is again observed to be the most stable.
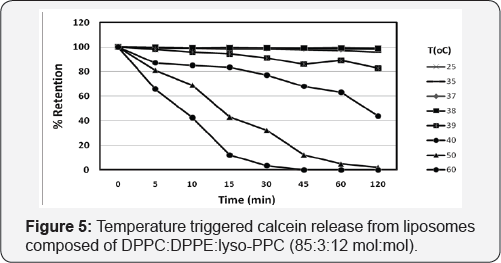
Size measurements of the encapsulating liposomes before and after incubation in changing concentrations of calcium chloride resulted in an increased liposome size for all the formulations studied. Small micelles of 8-15 nm started to dominate in the case of 6% DPPE at increased concentration of Ca 2+ due to the dissociation of the DPPE from the lipid membrane, resulting in lower stability and rapid calcein leakage. Supporting these results, Peschkaa et al. [16] previously demonstrated that liposomes composed of phospholipids with -PE head group are more sensitive to serum proteins and calcium than -PC phospholipids due to a phase transfer from liquid crystalline to hexagonal.
Temperature-Triggered Calcein Release from DPPC: DPPE: lyso-PPC liposomes (85:3:12 mol/mol)
Both DSC and release studies indicated that liposomes of 3&; were more stable in the presence of increased serum and Ca2+ concentrations than the other liposome formulations studied, Since the main objective of this report is to demonstrate the curvature tuned preparation method for the preparation of thermosensitive liposomes for drug delivery, the efficiency of the thermosensitive liposomes to release the encapsulated calcein at elevated temperatures was evaluated. As illustrated in Figure 5, an increase in temperature from 37 °C to 39 °C resulted in the release of 20° of the calcein from the lipids after 2 hours, while further increasing the temperature to 40°C lead to a more rapid leakage of up to 60% of the encapsulated calcein. At even higher temperatures, 50 °C and 60 °C a very rapid release of almost all of the encapsulated calcein was achieved in 120min and 30min, respectively, whilst almost no release was observed at temperatures below 38 °C, highlighting the liposome stability and applicability to controlled release and clearly demonstrating that the liposomes prepared in the absence of any solvent using the curvature tuned liposome preparation method, can be triggered to release their contents by increasing the temperature (in a mild range), which is comparable to the temperaturesensitive liposomes reported by Needham [16].
Conclusion
Thermo-sensitive liposome formulations of DPPC:DPPE: lyso PPC with changing DPPE mol % were studied for their potential use as controlled drug release systems. A decreasing liposome size with increasing concentrations of DPPE was observed as a result of the truncated cone shaped DPPE providing a higher membrane curvature. Furthermore, an increased melting temperature (TM) and heat capacity (Cp) of liposomes was obtained with liposome formulations prepared with 3% of DPPE as compared with 1.5% indicating the incorporation of DPPE molecules with a melting temperature around 60 °C to the membrane and increased stability of those membranes compared to that of other formulations. When the DPPE concentration was increased to 6% both the TM and Cp decreased, which can be attributed to a disorganized membrane due to the incorporation of a higher amount of DPPE. In the presence of CaCl2, an increased calcium concentration leads to decreased membrane stability, and no transition peak which relates was observed after 0.4 mM of CaCl2 for 1.5% DPPE and 1.2 mM for 6% DPPE liposomes, whilst the 3% DPPE liposome formulation was shown to be more stable at high concentrations of calcium.
A critical factor to be studied when evaluating liposomes for in vivo drug delivery is the effect of serum on liposome stability. Again, the liposomes prepared with 3% of DPPE were observed to be more stable, when they incubated in the presence of 50% serum (blood concentration) than the other formulation studied. After 24hours of incubation, the liposomes were observed to have retained 73 and 45% of the encapsulated calcein, in the absence and presence of serum, respectively. In conclusion, the solvent-free curvature tunable preparation method was used to prepare thermosensitive liposomes for controlled drug release. The concentration of DPPE in the liposome formulation was found to be an important parameter and it affected the liposome size, release kinetics as well as the membrane integrity if liposomes of DPPC:DPPE:lyso-PPC. Liposomes of 3% DPPE were demonstrated to be thermo-sensitive, showing a temperature- dependent calcein release with increased release kinetics at temperatur higher than 38 °C, whilst being completely stable at physiological temperatures of 37 °C, and lower.
For More Articles in Global Journal of Nanomedicine
https://juniperpublishers.com/gjn/GJN.MS.ID.555579.php
Please Click on: https://juniperpublishers.com/gjn/index.php
For More Open AccessJournals In Juniper Publishers Please Click on: https://juniperpublishers.com/index.php
Comments
Post a Comment